Reliable production of plastic films and fibers will be crucial to many, many other aspects of the colony, including nutricyling, food production, synthesis of medicines and other useful chemicals, and construction of habitats. The following is a brief rundown of a few promising options.

Polyhydroxybutyrate (PHB) is a well-known and very promising bioplastic. Many species of bacteria (especially Bacillus cereus) can produce it with zero genetic modification or artificial selection. It is produced as granules inside the cytoplasm, with stress having the potential to increase this production to a significant percentage of their weight (30-50%).
I believe that by lysing the cells in a standard blender (or perhaps by freezing) and then centrifuging, these granules would likely separate into a visible layer and be easily extracted from the rest of the cell matter. Either as part of this extraction step, or for forming/3D printing, the granules could also be dissolved in chloroform or acetic acid. https://www.nature.com/articles/srep17884 The latter would be preferable as it's easier to produce biologically and not as toxic, but both are fairly simple.
PHB will likely need plasticizers mixed in to improve its flexibility and decrease brittleness, either as a film or as a 3D printed part. Vegetable oil (easily derived from crops or from algae) is one option, as is glycerol. Glycerol is a byproduct of the standard production of biodiesel from plant oils (The reaction of the oil along sodium hydroxide in a methanol solution), so might happen to be available if the colony used/produced biodiesel.
Polylactic acid is another well-known bioplastic, and one that's used even now in conventional 3D printers. Lactobacillus spp. are bacteria which naturally produce lactic acid during anaerobic digestion of carbohydrates. This includes sugars, more complex starches, and potentially even raw cellulose if it's pre-digested by other enzymes or chemical processes beforehand (e.g. alkalis similar to the process for creating wood pulp).
The bacteria and feedstock can be filtered from the solution, and the lactic acid precipitated out by the addition of calcium carbonate. This reacts to produce calcium lactate salts, and carbon dioxide. The calcium lactate is insoluble, and so drops out of solution and can be easily collected. The calcium lactate can be treated with dilute sulfuric acid (5%) to make calcium sulfate (insoluble) and lactic acid, so it's comparatively easy to separate a pure product. Sulfuric acid could be produced from the sulfur byproduct of a biodigester, though calcium carbonate is more difficult and regenerating it from the calcium sulfate is a challenging proposition. Potentially different bases could be used to precipitate out the lactic acid, which are more amenable to regeneration/continuing cycling.
After a purified lactic acid monomer is obtained, it must be polymerized into the polylactic acid. I believe the best approach is a direct polycondensation. A ‘ring opening’ polymerization is also possible after conversion of lactic acid to the diester lactide, but this is more complex and provides a lower molecular weight product. Direct polycondensation requires low temperatures in a dehydrating environment along with constant mixing and agitation, though temperatures must also be high enough to melt the PLA. One approach would be to use digital temperature control alongside a magnetic stirbar and a vaccuum pump. This removes water from the solution, gradually shifting the reaction equilibrium towards high molecular weight PLA. The higher the temperature, the more the diester lactide ring is formed as a byproduct, lowering final molecular weight and reducing yield slightly. 180*C is the approximate ideal.
Optionally, there is also solid-state polycondensation (SSP). After carrying out the above steps briefly, the PLA can be allowed to cool and solidify around 20-25*C below its melting point. At this point it is solid, but still capable of continuing the polycondensation reaction, increasing its molecular weight, sometimes by orders of magnitude. This is a long process however, often taking multiple days even with metal or metal salt catalysts (Sn, Zn). Perhaps it would be a necessary part of the manufacture, or would only be used when high molecular weights (causing higher density, greater strength, etc.) is necessary.
Rough protein/cellulose films are another option. Agricultural waste or any other product with cellulose and protein content can be treated with a number of simple acids and then pressed into shape, yielding a film with only low permeability to water and air. Formic acid (the simplest organic acid) could be used, and would be relatively simple to manufacture either by direct microbial synthesis or partial oxidation of methane into methanol, and then formic acid. This could be an option for low-stress, non-critical areas, such as flooring or containers for growing terrestrial plants, open storage containers, furniture, etc.
Nanocellulose is a highly promising material as well. Bacteria cellulose can be produced quickly, inexpensively, and yields a very pure product, even if other sources of cellulose (wood pulp, flax/hemp fibers) are unavailable. Though the current partial-oxidation used to produce nanoscale features on treated cellulose uses a complex aminoxyl radical (TEMPO), some researchers have had excellent luck using only nitric acid in conjunction with sodium nitrite. Nitric acid and sodium nitrite are readily produced from atmospheric nitrogen through N-fixing and N-oxidizing microbes.
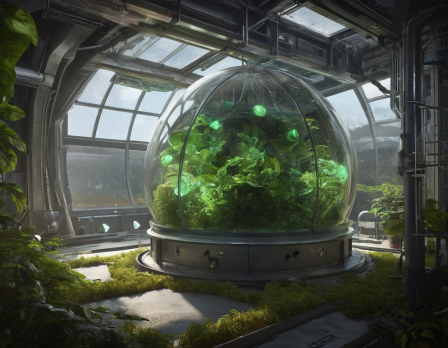